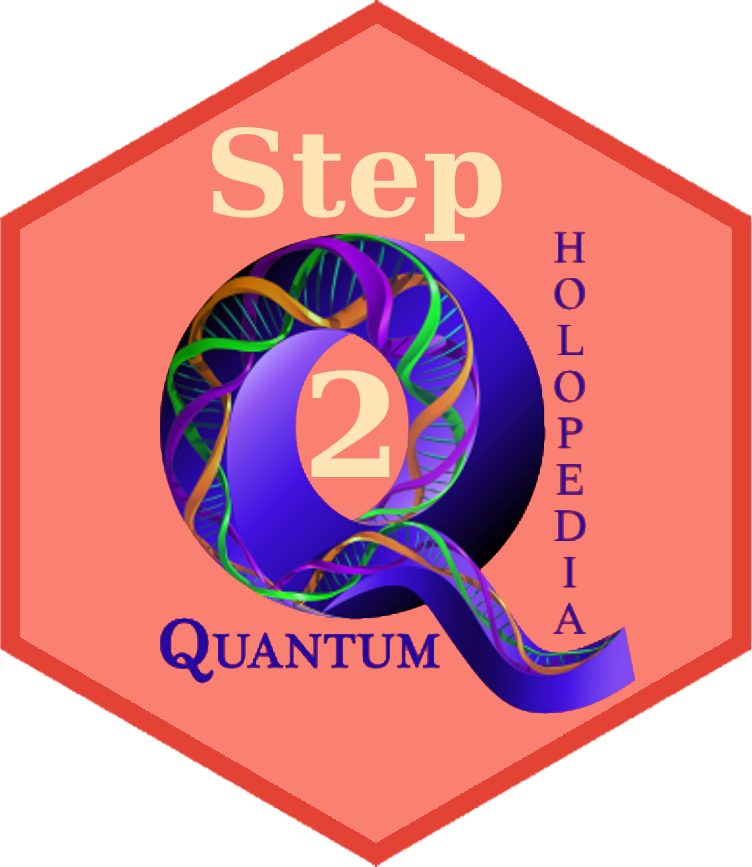
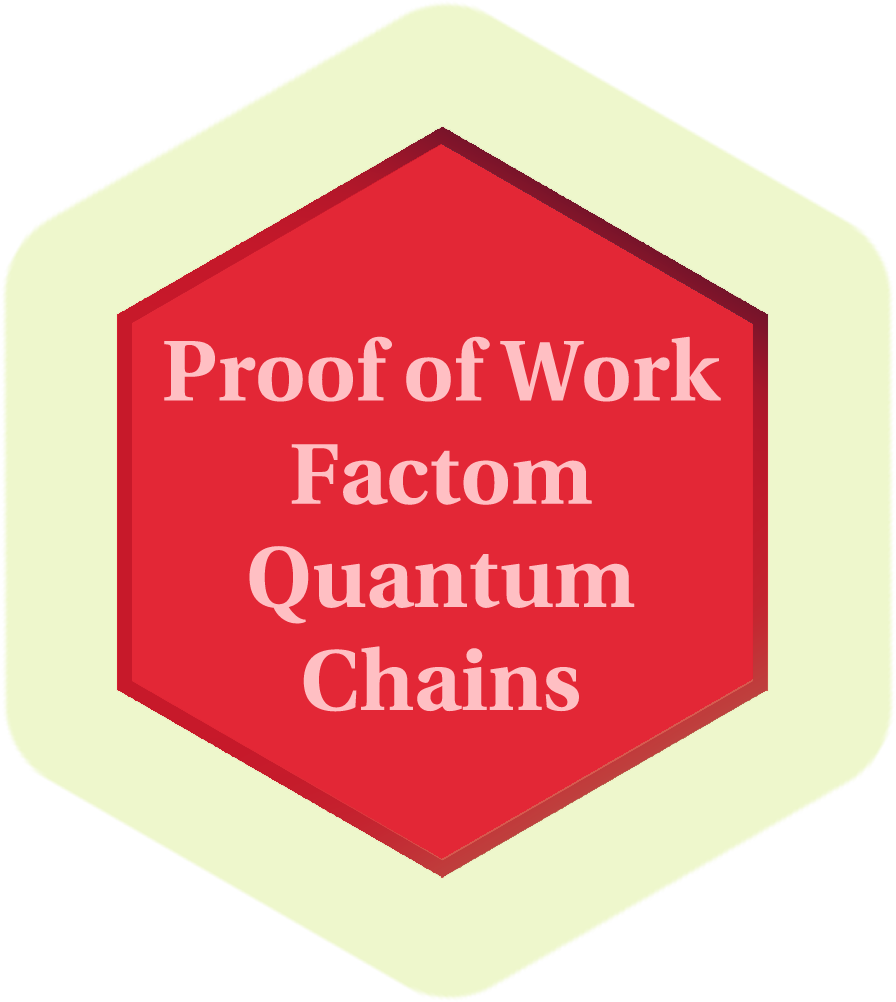
DARQ GPT ∞ Holon Coin
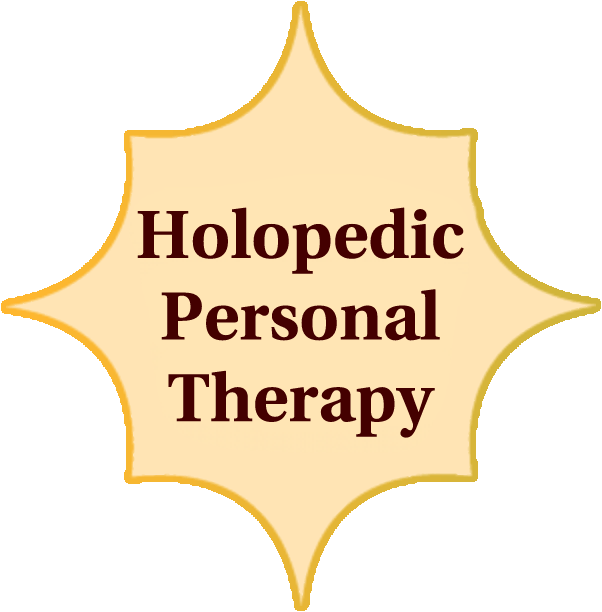
DARQ GPT ∞ Step 2 ∞ Analitics DARQ tchnology ∞ Proof of Work Holon Quantum DARQ with DARQ GPT
The next step is to apply Quantum Artificial Intellect to business projects. DARQ GPT creates a Holon (Holographic Operating Network) quantum network simulation in a distributed database managed in a peer-to-peer communication model using technologies such as Bitcoin, Ethereum and Factom Qtum’s dLoc by SMARTRAC [1, 2]. Documents and files obtained in Consortium Quanticum projects, such as the results from TimeWaver Med/Timewaver Frequency, fMRI EEG, MEG, PET 3D tomography, fMRI/EEG, MEG/EEG, fNIRS/EEG [3], are transmitted and processed on these networks and will be used as an analytical tool for big data and machine predictive learning. The purpose of this analysis is to detect anomalies in the area of quantum oscillations and to develop a statistical association of these anomalies for events observed in media, f.ex. social media interpreted using sentiment analysis quantum algorithms.
Predictive holo diagnostic data through quantum algorithms based deep learning algorithms and Factom Quantum Chain technology
DARQ GPT ∞ Rare and abundant retrospective data for predictive analysis, biobank & personalized medicine
Large amounts of data being produce by the medical industry require growing infrastructure to store and manage it in a secure manner. At the same time, these vast collections of data provide huge potential for interpretation and predictive analysis with the use of the right tools. Answers to those demands are well provided by Factom Quantum Chain technology and quantum algorithms inspired deep learning algorithms respectively.
Factom Quantum Chain technology can establish a decentralized personal health data ecosystem, something like a digital Biobank & personalized medicine, where medical data are stored and maintained, ready to be used by medical professionals for research and health monitoring and available for the patients who have the full control over the privacy settings of their data. Quantum teleportation is used to transmit a quantum qubit coin [1].
Quantum Algorithms inspired by Deep Learning Algorithms, e.g. Quantum Neural Networks ∞ QNN and Quantum Fuzzy Logic ∞ QFL, can convert simple data into powerful sources of data for predictive analytics f.ex. by using combination ∞ quantum kinematic ∞ relationship value of the data. Data optimized for the use in Quantum Algorithms inspired Deep Learning Algorithms and to be displayed as holographic visualizations is acquired from various measurements such as basic blood test, basic urine test, Holo MRI, Holo EPR / NMR, MEG, Holo fNIRS, Holo EEG, Holo ECG, genome, transcriptome, microbiome, etc. and their combinations have relevant value for the certain disease condition. The main categories the data can be divided into are: dynamic 4D ∞ which reflects the organism’s state at the time of sampling (blood test, transcriptome, epigenome, proteome, microbiome, etc.) and containing both rapidly and gradually changing data, and static 3D ∞ that remains mostly unchanged during the patient’s life (genome, fingerprint) [2]. There are various simulators that can simulate quantum computations on classical computers [3]. They can serve as platforms to design test quantum algorithms that will be later translated into their classical equivalents.[1] K. Ikeda, “qBitcoin: A Peer-to-Peer Quantum Cash System,” in Advances in Intelligent Systems and Computing, Springer International Publishing, 2018, pp. 763–771.[2] P. Mamoshina et al., “Converging blockchain and next-generation artificial intelligence technologies to decentralize and accelerate biomedical research and healthcare,” Oncotarget, vol. 9, no. 5, Nov. 2017.[3] List of QC simulators on Quantiki
DARQ GPT ∞ Nervous System in quantum entanglement
DARQ GPT ∞ Synaptic Quantum Tunnelling in Brain Activity
DARQ GPT ∞ Quantum Parallel Transference
DARQ GPT ∞ It is highly probable that the clock
frequencies of 16 MHz,
75 MHz, 333 MHz and 1400 MHz are Proton-resonance-frequencies.

DARQ GPT Tunnel Holotomography
Holographic representation of quantum and spacetime dimensions entangled through gravitoelectromagnetism, and whose correlation is available in real time during increased states of brain activity.
Scanning Tunnels Tomography
Scanning Tunnel Tomography is based on knowledge in quantum and holographic physics as well as on gravitoelectromagnetism. Based on the quantum knowledge, we generate and simulate holographic and 4D representations that are correlated with quantum phenomena. The essence is to include quantum phenomena into certain dynamic symbols of computer holography representing the activity and morphology of the brain as well as the anatomy and morphology of the body. The images are created based on readings of dynamic waveforms of electromagnetic pulses during scanning. They are different from standard electrical scanning devices, where we can make a feedback loop, as is in the case of biofeedback. In biofeedback, we give a negative feedback signal, from the body or from the brain, and we modify the results based on the information about the system. In quantum systems, it cannot be done because the observer’s energy, which biofeedback is as well, strains the system. We create holographic simulations and based on them we build parallel information on quantum states. It is not known at this stage what is the cause and what is the effect. At this stage, the concept of the flow of parallel information, the quantum dimension and its holographic analog, are created, which are not constricted, i.e. they do not burden each other, in other words, they are remodeled according to the principles of quantum holographic superposition. This allows training using information from the quantum system, which will travel to the results in the holographic system, which is generated simulation, just as the 3D image is compared with the mapping based on conductance readings from the electromagnetic waveforms of the brain. The essence is that the quantum phenomena are incorporated into certain holographic symbols. It may also be the opposite, so that holographic symbols can be introduced into quantum processes, e.g. by creating appropriate visualizations that can create holographic quantum reality through appropriate states, e.g. strong emotional arousal. The direction of the influence of one system on the other is not assumed, since it is not a direct influence (for example an influence on the principle of molecular interaction of electrons, etc.) as in the case of biofeedback. Instead, these flows are on the basis of entanglement, that is, the effect of transferring the state of spins from one layout to another. The possibility of interacting holographic and quantum systems is assumed and the direction of information flow is secondary.
Projects such as The Virtual Brain TVB provide tools to create simple yet realistic brain models coherent with clinical brain scanners [4].
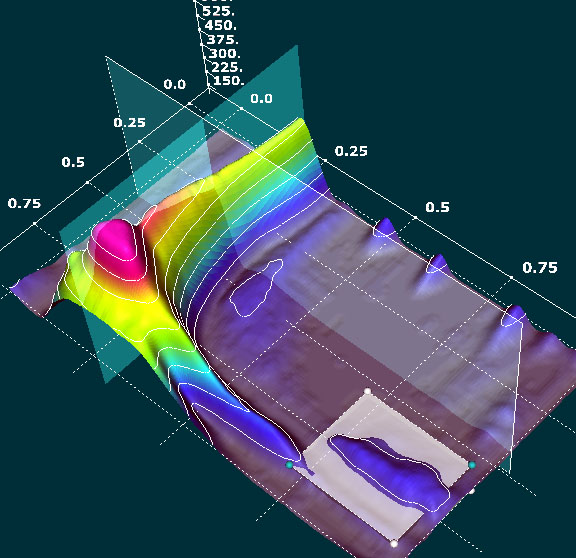
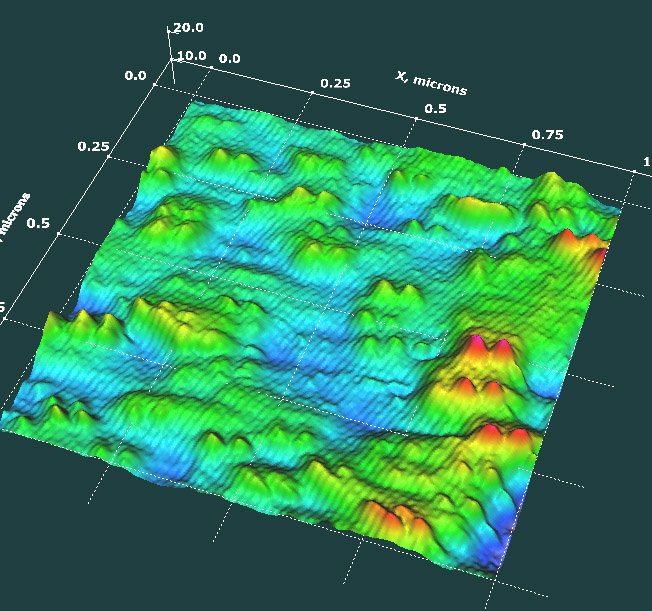
3D images, holograms and bioholograms
Waveforms recorded in this way are interpreted and transformed into putative 3D images, holograms, bioholograms and maps, which are closer to the present understanding of the nature of a given phenomenon in space-time. This transformation is done using the iterative learning method, which involves the selection of parameters at each of its steps so that the representation of the results obtained agrees with the current level of knowledge about the scanned phenomenon. And so EEG scans serve to obtain 3D images, holograms and maps of brain processes and brain morphology, modeled on images obtained using parallel cognitive approaches such as fMRI, CAT, or computerized brain tomography. Further, using MRI the whole body can be scanned and transformed into biohologram body. Quantum algorithms for processing EEG measurements for holographic representations are created based on the repeatability of the obtained representations in regards to defined variable patterns obtained from above mentioned parallel techniques.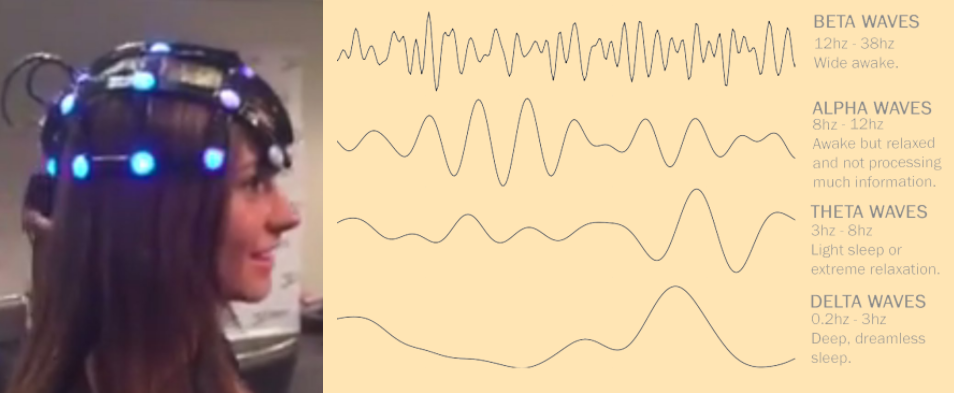
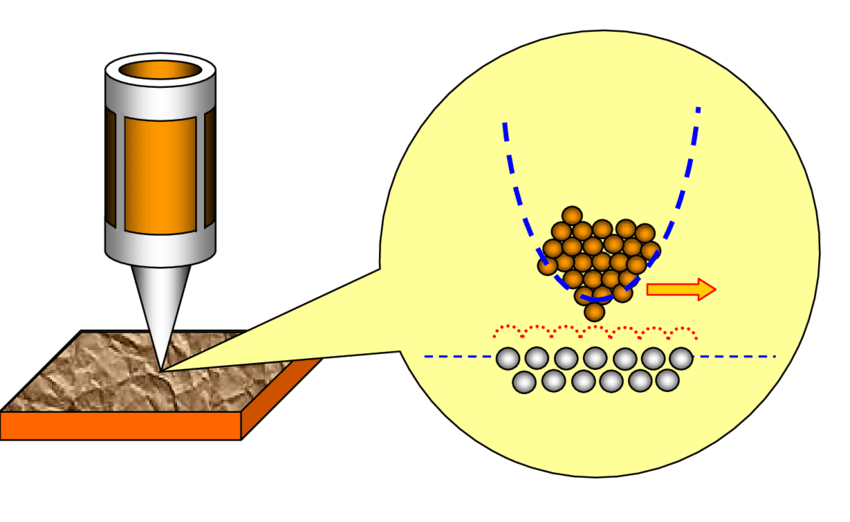
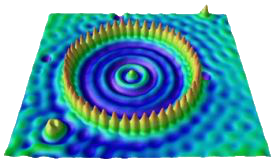